Introduction
Liquid cold plates, a cornerstone of industrial cooling solutions, are pivotal in maintaining optimal temperatures for various processes and equipment. Within this landscape, KenfaTech stands out as a leading provider of innovative cooling solutions. These plates are not mere components but integral systems designed to efficiently dissipate heat, ensuring the seamless operation of critical machinery.
Fluid dynamics emerges as a key protagonist in the narrative of heat transfer efficiency within liquid cold plates. The interplay of fluid behavior, governed by parameters such as velocity, pressure, and turbulence, dictates the efficacy of heat dissipation. In this realm, KenfaTech's commitment to excellence shines through, with a deep understanding of how fluid dynamics influences the performance of its liquid cold plates.
This article embarks on a journey to unravel the complex relationship between fluid dynamics and the efficacy of liquid cold plates, with KenfaTech's expertise guiding the way. By shedding light on the fundamental principles governing fluid flow within these systems, this exploration aims to empower potential buyers. Whether considering options from KenfaTech's range of offerings or contemplating custom solutions, a nuanced understanding of fluid dynamics is paramount for making informed decisions.
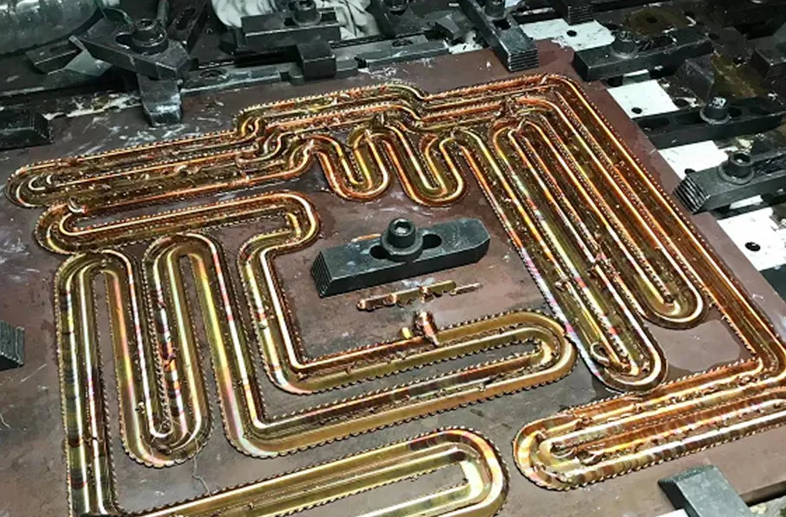
Fundamentals of Fluid Dynamics in Liquid Cold Plate Systems
Fluid dynamics within liquid cold plate systems govern the intricate interplay of various factors influencing heat transfer efficiency. Understanding these fundamentals is paramount for optimizing the performance of such systems.
Explanation of Fluid Flow Behavior
Within the channels of a liquid cold plate, fluid flow exhibits complex behavior influenced by channel geometry, fluid properties, and external factors. As fluid traverses the channels, it experiences frictional forces against the channel walls, leading to velocity and pressure distribution changes.
Key Parameters Affecting Fluid Dynamics
Several vital parameters significantly impact fluid dynamics within liquid cold plate systems:
- Velocity: The speed at which the fluid flows through the channels directly affects heat transfer rates. Higher velocities promote more excellent convective heat transfer.
- Pressure: Pressure differentials along the flow path drive fluid movement within the liquid cold plate. Proper management of pressure ensures uniform flow distribution and efficient heat dissipation.
- Reynolds Number: A dimensionless parameter representing the ratio of inertial to viscous forces, the Reynolds number characterizes flow regimes within the liquid cold plate. Understanding the Reynolds number helps predict flow behavior and transition between laminar and turbulent flow.
- Turbulence: Turbulent flow, characterized by chaotic and unpredictable fluid motion, enhances heat transfer compared to laminar flow. Turbulence promotes mixing and convective heat exchange, thereby improving cooling efficiency.
Importance of Laminar vs. Turbulent Flow Regimes
Choosing between laminar and turbulent flow regimes significantly impacts heat transfer efficiency within liquid cold plate systems. While laminar flow offers predictable behavior and lower pressure losses, turbulent flow facilitates higher heat transfer rates due to increased mixing and convective effects. Balancing these factors is crucial for optimizing cooling performance.
Impact of Fluid Properties on Heat Transfer Efficiency
The properties of the coolant used significantly influence the efficiency of heat transfer within liquid cold plate systems. Understanding how these fluid properties affect heat dissipation is crucial for optimizing cooling performance.
Influence of Coolant Properties
The viscosity, density, and thermal conductivity of the coolant play vital roles in determining its effectiveness in heat dissipation:
- Viscosity: The viscosity of the coolant affects its resistance to flow within the liquid cold plate channels. Higher viscosity fluids experience more excellent resistance, potentially leading to higher pressure losses and reduced flow rates. Conversely, lower-viscosity fluids facilitate smoother flow, enhancing heat transfer efficiency.
- Density: Density dictates the mass of the coolant per unit volume and influences its buoyancy and flow characteristics. Fluids with higher density exhibit greater thermal inertia, potentially resulting in slower temperature changes within the system. Understanding density variations is essential for maintaining uniform temperature distribution across the liquid cold plate.
- Thermal Conductivity: The thermal conductivity of the coolant determines its ability to conduct heat away from the heat source and distribute it evenly across the liquid cold plate. Coolants with higher thermal conductivity offer more efficient heat transfer, minimizing temperature gradients and maximizing cooling performance.
Selection Criteria for Optimal Coolant Type
When selecting the optimal coolant type for a liquid cold plate system, various application-specific requirements must be considered:
- Operating Conditions: The coolant must be suitable for the system's operating temperature range to ensure thermal stability and performance reliability.
- Compatibility: Compatibility with system materials and components is essential to prevent corrosion, degradation, or other adverse effects.
- Heat Dissipation Requirements: The coolant should possess sufficient heat dissipation capabilities to meet the cooling demands of the application, considering factors such as heat load and desired temperature regulation.
- Environmental Considerations: Environmental factors, such as toxicity, flammability, and environmental impact, must be considered to ensure safety and compliance with regulations.
Effects of Coolant Flow Rate and Temperature
The flow rate and temperature of the coolant significantly impact heat transfer performance within liquid cold plate systems:
- Flow Rate: Higher coolant flow rates promote more excellent convective heat transfer by increasing fluid velocity and enhancing mixing within the channels. However, excessively high flow rates may lead to increased pressure losses and reduced efficiency.
- Temperature: The temperature of the coolant affects its thermal properties and the extent of heat transfer. Proper temperature control is essential to maintain optimal operating conditions and prevent thermal degradation of system components.
Design Considerations for Enhancing Fluid Dynamics
Enhancing fluid dynamics within liquid cold plate systems is essential for optimizing heat transfer efficiency. Several design considerations are crucial in promoting efficient fluid flow and maximizing cooling performance.
Importance of Channel Geometry and Layout
The geometry and layout of channels within liquid cold plates significantly impact fluid flow patterns and heat transfer capabilities:
- Channel Dimensions: Optimizing channel dimensions, including width, depth, and spacing, influences fluid velocity and pressure distribution. Appropriately sized channels facilitate uniform flow and minimize pressure losses.
- Channel Orientation: The orientation of channels relative to the heat source affects heat dissipation efficiency. Directing flow perpendicular to the heat source maximizes convective heat transfer, while parallel flow minimizes pressure drop.
Role of Flow Distribution Enhancements
Flow distribution enhancements such as manifolds and flow straighteners play a vital role in promoting uniform flow distribution and minimizing flow maldistribution:
- Manifolds: Manifolds facilitate the even distribution of coolant flow to individual channels within the liquid cold plate. Properly designed manifolds ensure balanced flow rates and prevent stagnation zones, optimizing heat transfer efficiency.
- Flow Straighteners: Flow straighteners mitigate disturbances and turbulence, promoting laminar flow and minimizing pressure losses. By smoothing flow profiles, flow straighteners enhance heat transfer performance and reduce the risk of flow separation.
Integration of Advanced Features
The integration of advanced features like microchannels offers additional opportunities for enhancing heat transfer performance:
- Microchannels: Microchannels, characterized by small hydraulic diameters and high surface area-to-volume ratios, facilitate efficient heat transfer through enhanced convective effects. Microchannels promote turbulence and increase the surface contact area, enabling higher heat fluxes and improved cooling efficiency.
Custom liquid cold plates tailored to specific application requirements can leverage these design enhancements to achieve superior cooling performance across diverse industrial applications.
Practical Implications and Performance Evaluation
Understanding the practical implications of fluid dynamics optimization and conducting thorough performance evaluations are essential steps in maximizing the effectiveness of liquid cold plate systems.
Comparative Analysis
Comparative analysis allows for a systematic comparison of different liquid cold plate designs to assess fluid dynamics efficiency:
- Design Comparison: Various liquid cold plate designs, from traditional finned designs to advanced microchannel configurations, are evaluated based on fluid flow characteristics and heat transfer performance.
- Fluid Dynamics Metrics: Comparative analysis considers metrics such as pressure drop, flow uniformity, and heat transfer coefficient to quantify the efficiency of different designs in promoting effective fluid dynamics and heat dissipation.
Evaluation Methods
Methods for evaluating and quantifying heat transfer efficiency in industrial settings provide valuable tools for assessing liquid cold plate performance:
- Experimental Testing: Conducting practical tests using prototype liquid cold plates allows for directly measuring heat transfer rates and fluid flow characteristics under controlled conditions.
- Computational Fluid Dynamics (CFD): CFD simulations enable a virtual analysis of fluid dynamics within liquid cold plate systems, offering insights into flow patterns, temperature distribution, and pressure drop without physical prototypes.
- Performance Metrics: Performance metrics such as thermal resistance, thermal conductivity, and overall heat transfer coefficient provide quantitative measures of heat transfer efficiency, aiding in comparing different liquid cold plate designs and configurations.
By leveraging case studies, comparative analysis, and evaluation methods, liquid cold plate suppliers and buyers can understand the practical implications of fluid dynamics optimization. These insights inform the selection of custom liquid cold plates tailored to specific application requirements, ultimately maximizing cooling performance in industrial settings.
Challenges and Future Directions
Navigating the challenges and exploring future directions in optimizing fluid dynamics within liquid cold plate systems is essential for advancing cooling technology and meeting evolving industrial demands.
Addressing Common Challenges
Optimizing fluid dynamics within liquid cold plate systems presents several challenges:
- Flow Maldistribution: Uneven flow distribution can lead to localized hotspots and reduced cooling efficiency. Addressing flow maldistribution requires careful design considerations and flow control mechanisms to ensure uniform coolant distribution.
- Pressure Drop: High-pressure losses within liquid cold plate channels can limit flow rates and compromise overall system performance. Minimizing pressure drop while maintaining adequate heat transfer rates requires optimization of channel geometries and flow distribution mechanisms.
- Heat Transfer Enhancement: Despite advancements in understanding fluid dynamics, further enhancing heat transfer efficiency remains challenging. Exploring novel heat transfer enhancement techniques like surface modifications and nanofluids may unlock new opportunities for improving cooling performance.
Emerging Trends and Innovations
Emerging trends and innovations in fluid dynamics research are shaping the future of liquid cold plate technology:
- Advanced Materials: Developing novel materials with tailored thermal and hydraulic properties enables the design of more efficient liquid cold plates. Advanced materials offer enhanced heat transfer capabilities while maintaining compatibility with harsh operating conditions.
- Computational Tools: Continued advancements in computational fluid dynamics (CFD) modeling and simulation techniques facilitate virtual prototyping and optimization of liquid cold plate designs. CFD enables rapid iteration and exploration of complex flow phenomena, accelerating innovation in cooling technology.
- Multi-Physics Integration: Integrating multiple physical phenomena, such as fluid flow, heat transfer, and structural mechanics, allows for a holistic approach to liquid cold plate design. Multi-physics simulations enable comprehensive optimization of system performance while considering various interacting factors.
Potential Advancements in Technology
Future advancements in liquid cold plate technology driven by fluid dynamics insights hold promising prospects:
- Intelligent Cooling Systems: Integration of sensors and actuators enables real-time monitoring and control of coolant flow and temperature within liquid cold plate systems. Intelligent cooling systems adapt dynamically to changing operating conditions, maximizing efficiency and reliability.
- Microscale Manufacturing: Advancements in microscale manufacturing techniques enable the fabrication of intricate liquid cold plate structures with precise control over channel geometries and surface properties. Microscale features enhance heat transfer performance and enable compact, high-performance cooling solutions.
- Hybrid Cooling Approaches: Combining liquid cooling with other cooling technologies, such as phase-change materials or thermoelectric cooling, offers synergistic benefits for temperature regulation and heat dissipation. Hybrid cooling approaches leverage the strengths of different cooling mechanisms to achieve optimal thermal management.
By addressing common challenges, embracing emerging trends, and exploring potential advancements, the liquid cold plate industry can continue to evolve and innovate, driving toward more efficient and reliable cooling solutions for diverse industrial applications.
Conclusion
Fluid dynamics is a paramount factor in shaping the efficiency and efficacy of liquid cold plate systems for heat transfer applications. Throughout this discourse, the profound influence of fluid behavior within these systems, particularly in optimizing heat dissipation, has been underscored.
For potential buyers evaluating cooling solutions, particularly those offered by KenfaTech, it is imperative to grasp the significant impact of fluid dynamics on system performance. Factors such as channel geometry, flow distribution, and coolant properties are pivotal in determining heat transfer efficiency. By conscientiously considering these aspects, buyers can make well-informed decisions when selecting custom liquid cold plates tailored to their specific cooling needs.
As technology progresses and industrial requirements evolve, the necessity for continuous exploration and consultation with experts in fluid dynamics and heat transfer becomes increasingly apparent. Through collaboration with KenfaTech and other industry-leading liquid cold plate suppliers, businesses can leverage expertise to optimize their cooling solutions, achieving maximum efficiency and effectiveness in industrial applications.
In summary, fluid dynamics epitomizes the cornerstone of liquid cold plate technology, driving innovation and elevating system performance. By recognizing its paramount importance, embracing key takeaways, and actively engaging in collaboration, businesses can ensure the selection of optimized liquid cold plates, thereby enhancing their cooling capabilities and bolstering operational excellence.